Effect of phospholipid-containing eye drops on the de-wetting behaviour of soft contact lenses
Purpose: The primary objective of the study was to determine differences between in vitro surface de-wetting of soft contact lens (SCL) materials out of saline solution, before and after treatment with phospholipid containing rewetting drops.
Method and Materials: Fifteen lenses of different SCL material classes according to ISO18369 were tested (polymacon, nelfilcon A, ocufilcon D, balafilcon A, somofilcon A, lotrafilcon B). The lenses were rinsed and stored in saline solution to minimize the effect of the original blister solution. A de-wetting video was captured for every lens using the Keratograph 5M. Afterwards all lenses were soaked in phospholipid containing rewetting solution for 25 hours. The lenses were gently agitated by an orbital shaker with a frequency 0.25 Hz, tilting up to 15°. After treatment, all lenses were equilibrated again in saline solution for at least 30 minutes. De-wetting videos were captured again. In vitro “Non-Invasive Keratograph Dry Up Time“ (NIK-DUT) was assessed for a measurement time of 180 s. The results were expressed by the Area under Curve (AUC) as well as clinical relevant time point to reach 4.82 mm2 de-wetting.
Results: The AUC results before and after treatment with phospholipid containing rewetting solution were: polymacon 4870.9 ± 942.7 and 6214.1 ± 932.1, nelfilcon A 4688.0 ± 1065.1 and 6959.8 ± 1081.8, ocufilcon D 3612.6 ± 875.5 and 7042.0 ± 714.3, balafilcon A 4170.4 ± 927.2 and 7385.6 ± 879.8, somofilcon A 4284.50 ± 833.8 and 6762.2 ± 1380.2 and lotrafilcon B, comfilcon A 4973.3 ± 1249.3 and 7415.7 ± 994.8. All differences were statistically significant at a significance level of 0.05 with P<0.001 (Wilcoxon rank sum test). The mean time to reach 4.82 mm2 de-wetting was 19 s.
Conclusion: The used phospholipid containing solution did interact with all soft contact lens materials and lead to accelerated in vitro de-wetting. As the de-wetting area of 4.82 mm2 is reached after a typical interblink period for contact lens wearers during attentional visual tasks, it is unlikely to expect a drop of the visual acuity by one log line.
Introduction
The comfortable wear of contact lenses is always associated with a hydrophilic surface material.1 In addition, a wetted surface allows for a higher visual acuity than a partially dried contact lens surface.2 It is therefore of great importance to assess the tear film stability in clinical practice.3,4 The administration of artificial tears is recommended and common practice in the event of subjective symptoms such as dry eyes, fluctuating visual quality or burning eyes due to lack of tears or tear film instability.5 Artificial tears containing hyaluronic acid are a prominent treatment for such symptoms.6-9 These are often also approved for use when wearing contact lenses.10, 11 The age-related increase in dry eye symptoms is most often due to changes in the composition of tears. There is a causal relationship with regard to the secretion produced by the Meibomian glands, which is subject to changes due to the hyperkeratinisation of the squamous epithelium of the lid margin.12-14 The use of lipid-containing artificial tears makes sense17-19 in addition to therapeutic methods of lid cleaning and hygiene.15, 16 Some phospholipid-containing eye drops are also approved for use with contact lenses.20, 21 Since it is known that the dominant group of today’s CL materials, namely silicon hydrogel materials,22-24 are more lipophilic than hydrogel materials, the question arises as to how the phospholipid-containing constituents in an artificial tear solution affect the stability of the surface wetting. The term wetting is widely used, since numerous methods have been established to investigate the in vitro wetting of contact lenses (CLs). These include the Sessile Drop method,25, 26 the Captive Bubble method,27-29 and the Wilhelmy Plate method.30 These tests evaluate the tendency of a liquid to spread when it reaches a material surface. However, they can also be used for the reverse case, i.e. the evaluation of the drying behaviour of a surface. Video keratoscopy methods are also used in this context.31-34 Considering the de-wetting of a contact lens surface makes it possible to take into account any existing kinetic effects. The time-dependent stability of a thin liquid film is clinically relevant, since its instability can have a negative influence on vision.2 In addition to the visual quality, good wetting is also relevant for comfort. A surface with reduced wetting results in higher friction.35,36 A better wetted contact lens surface can therefore achieve a potentially better wearing comfort and have a positive influence on vision.37
Aim of the study
The primary objective of this study was to determine the de-wetting behaviour of different soft CLs which had previously been equilibrated in a standard saline solution or a phospholipid-containing solution. Furthermore, it should be investigated whether a clinically relevant de-wetting of the contact lens surface of 4.82 mm2 occurred in the typical period between two blinks2 if the contact lens material was previously in contact with a phospholipid-containing solution for a relatively long period of time.
Materials und Methods
A videotopograph (Oculus Optikgeräte GmbH, Keratograph 5M) (Figure 1) was used to investigate the de-wetting of the contact lens surface in vitro. The "non-invasive Keratograph dry up time" (NIK-DUT) is measured by projecting a Placido ring pattern onto the contact lens surface and then recording the reflected ring pattern.34 The wetting of the surface is regarded as stable if the reflection of the ring pattern is homogeneous. When the de-wetting process begins, small dents appear in the reflected ring pattern, which indicate a dilution of the liquid layer on the surface, followed by stronger distortions and bifurcations of the ring reflections if the boundary between the wet and dry surface is considered.34 In order to analyse a representative range of contact lens materials on the market, we selected one contact lens product per material class in accordance with the material class classification of the norm ISO 18369-1. Three charges of each contact lens product were procured, which comprised the vertex powers -3.0 D; +3.0 D and -6.0 D. Five CLs were investigated per vertex power, so that a total of 15 CLs were considered per material. 15 CLs per material were required, since the measuring process involved a manual transfer of the CL from the test liquid to the measuring station. The speed of this manual transfer can potentially change the thickness of the thin liquid layer, which can lead to fluctuations in the measurement result. Each recorded de-wetting video lasted 180 s. This period of time is sufficient for the lens surface to de-wett almost completely. Since the de-wetting occurs more quickly on the eye31,33 than in an in vitro setting, greater attention was paid to the de-wetting in the first seconds, until a dry area of 4.82 mm2 was reached, in order to better discuss the clinical relevance. The following methodology was applied in preparation for the NIK-DUT measurement: 12-well plates were filled with 2 ml of standardised saline solution with a pH of 7.4. The CLs were removed from the blister using tweezers. Care was taken to ensure that the tweezers did not touch the central measuring surface.28 The CLs were rinsed for 10 s from each side with standardised saline solution and then stored for 24 hours in this saline solution to equilibrate them. The samples were prepared by an unmasked examiner.
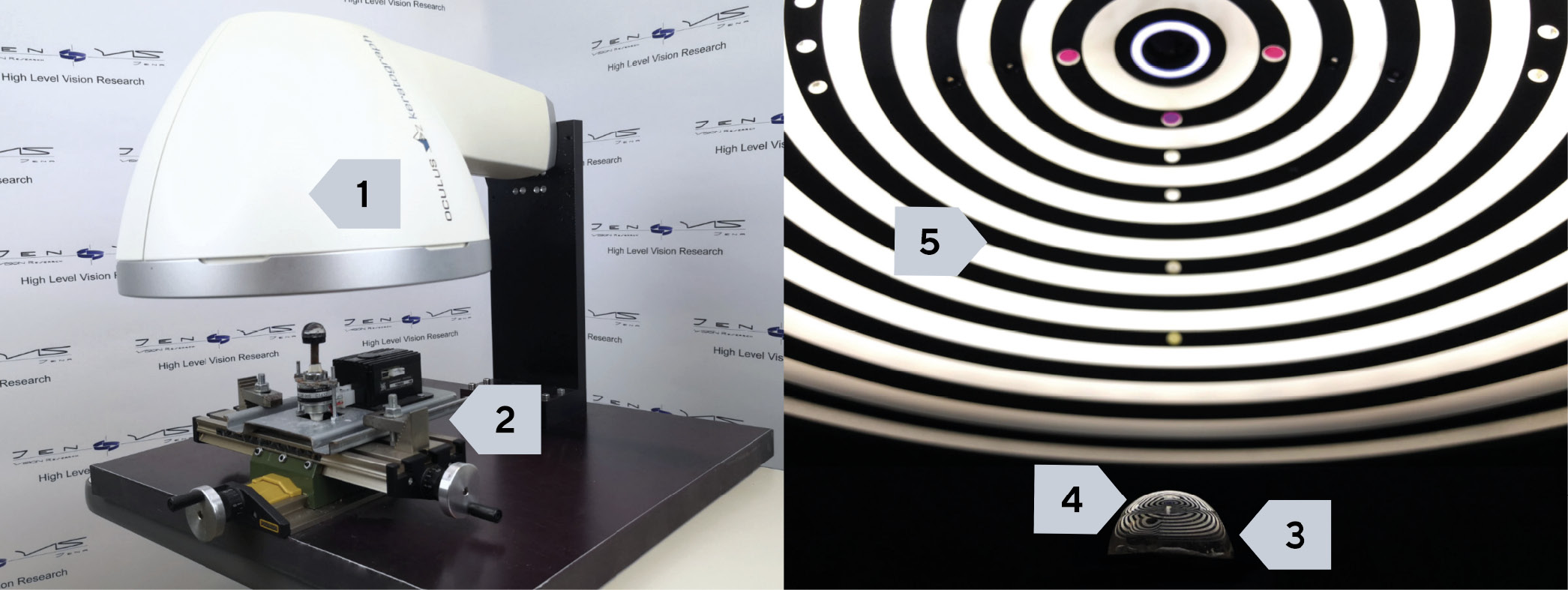
Subsequently, the de-wetting properties were determined by a masked examiner after the CLs had been stored in saline solution. For this purpose, the height of the lens support below the horizontally mounted K5M was set by means of the x-y-z coordinate table in such a way that a sharp image was obtained. The CL to be analysed was placed on the lens support from the saline solution and a 180 s long video was recorded with the NIK-BUT method using the software provided by the manufacturer. The CL was then transferred into well plates prepared with a phospholipid-containing solution (Hylex® with ectoin, soybean lecithin, physiological buffer, vitamin A and E, water; manufacturer: Bitop AG). The following "worst case" approach was used to mimic the typical contact time between the contact lens material and the phospholipid-containing solution as an in vitro test, based on everyday clinical practice. It was intended to simulate the use of artificial tears during one month. For this purpose, it was assumed that the drops were administered five times per day and that they stayed in the eye for ten minutes. The cumulative time in contact with the full concentration of the preparation was thus 25 hours, irrespective of the permitted period of use of the respective contact lenses. During this time, the well plates were placed on an orbital shaker, which tilted ± 15° in 15 cycles per minute.38 This procedure allowed a certain movement of the molecules of the artificial tear solution over the lens surface, which, in a simplified sense, reflects a simulation of the blink of the eye. In this way, a simulation of an in vivo situation was conducted. Following this phase, the CLs were transferred into standardised saline solution, in which they were stored for at least 30 minutes before the NIK-DUT measurement was carried out again. The videos were then analysed. For this purpose, a virtual grating consisting of 192 segments was placed on the contact lens surface to be measured, which represents a diameter of approximately 10 mm.34 An examiner, who had been instructed in the methodology, marked the surfaces which showed de-wetting. This was done for every second of the three-minute video.2 This close examination allowed the time-related bias of the actual drying to be kept as low as possible until de-wetting was actually detected in the video.
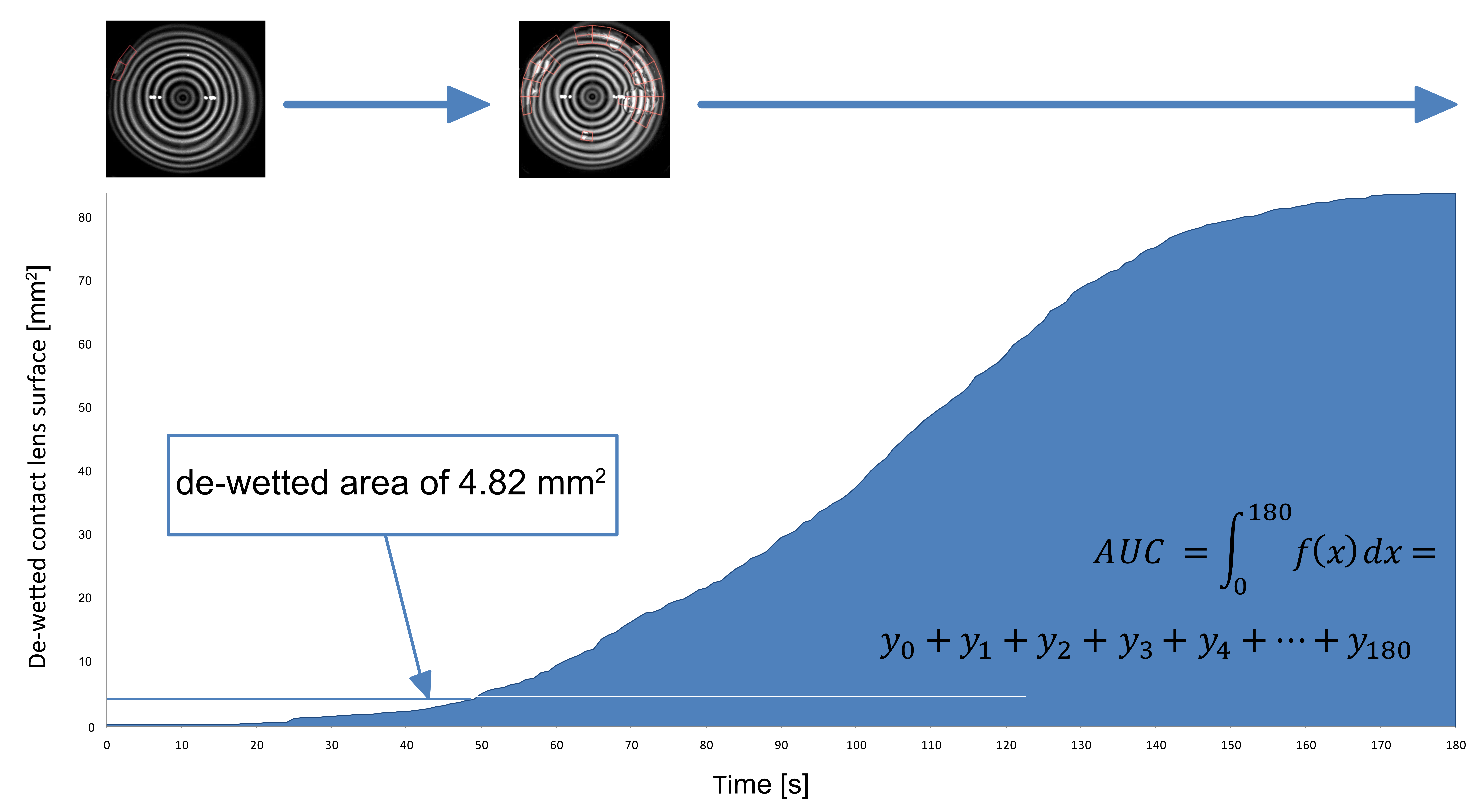
Statistical analysis
The data of the edited NIK-DUT measurements was output into a CSV table. Initially, the 15 CL for each material were grouped together. An analysis of the combined samples was carried out using the Wilcoxon rank-sum test (SPSS, version 25) for the following target variables:
Area under curve (AUC) over the measuring period of 180 s
As shown in Figure 2, the dynamic de-wetting process over the entire measuring period was taken into account. To observe the complete drying, approximately 180 s are required. Since each of the 192 segment fields is linked to a contact lens-related area indication, the dried area can be determined as a function of time. This is the basis for determining the AUC value.
Time to reach a de-wetted area of 4.82 mm2
A drop in visual acuity of one log level is expected in vivo with a de-wetted area of around 4.82 mm2. This dried surface does not necessarily have to be exactly central, since even medium-peripheral de-wetting can reduce the visual acuity due to scattered light effects.2
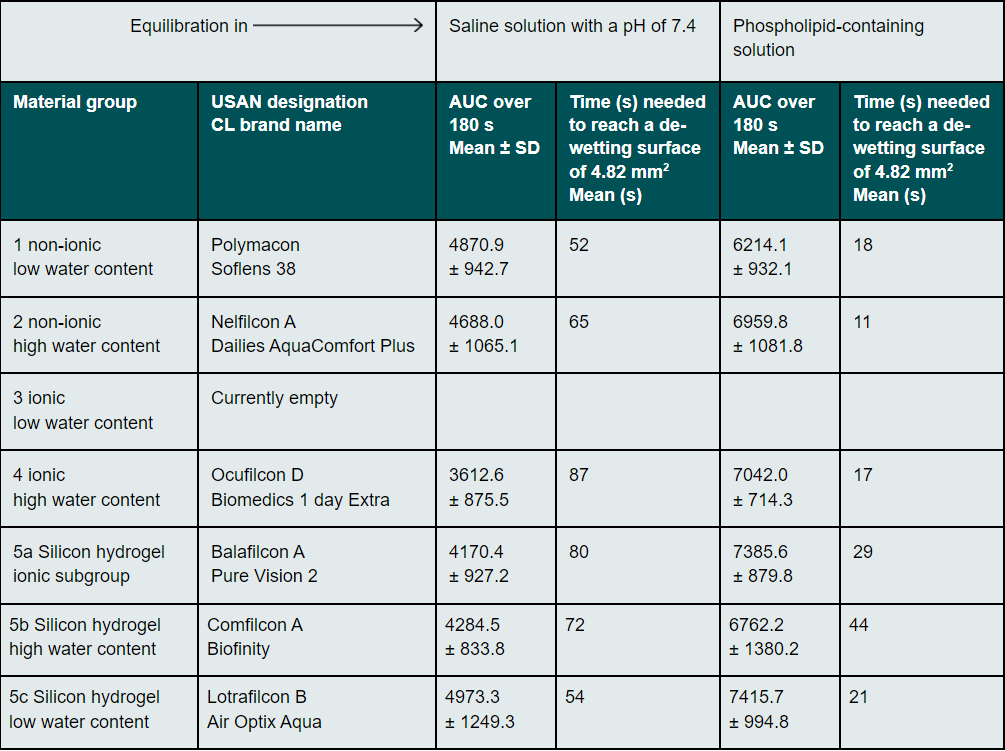
Results
The results for each material and both examination parameters are listed in Table 1. After storage in a phospholipid-containing solution, all contact lens materials dried up more rapidly and reached a de-wetting area of over 4.82 mm2 earlier (Wilcoxon rank-sum test, p < 0.001). Figure 3 shows this clearly. The blue-coloured curves represent the averaged and material-specific de-wetting observations over the measuring period of 180 s with regard to the de-wetted surface before using the phospholipid-containing solution. The green curves show that de-wetting took place faster after storage. On average, a dried area of 4.82 mm2 was achieved after 67 s for lenses stored in saline solution and after 19 s for those stored in a phospholipid-containing solution. This is clearly illustrated in Figure 4. The mean area determined by Kolbe et al. which leads to a decrease in visual acuity is reached after 19 s.
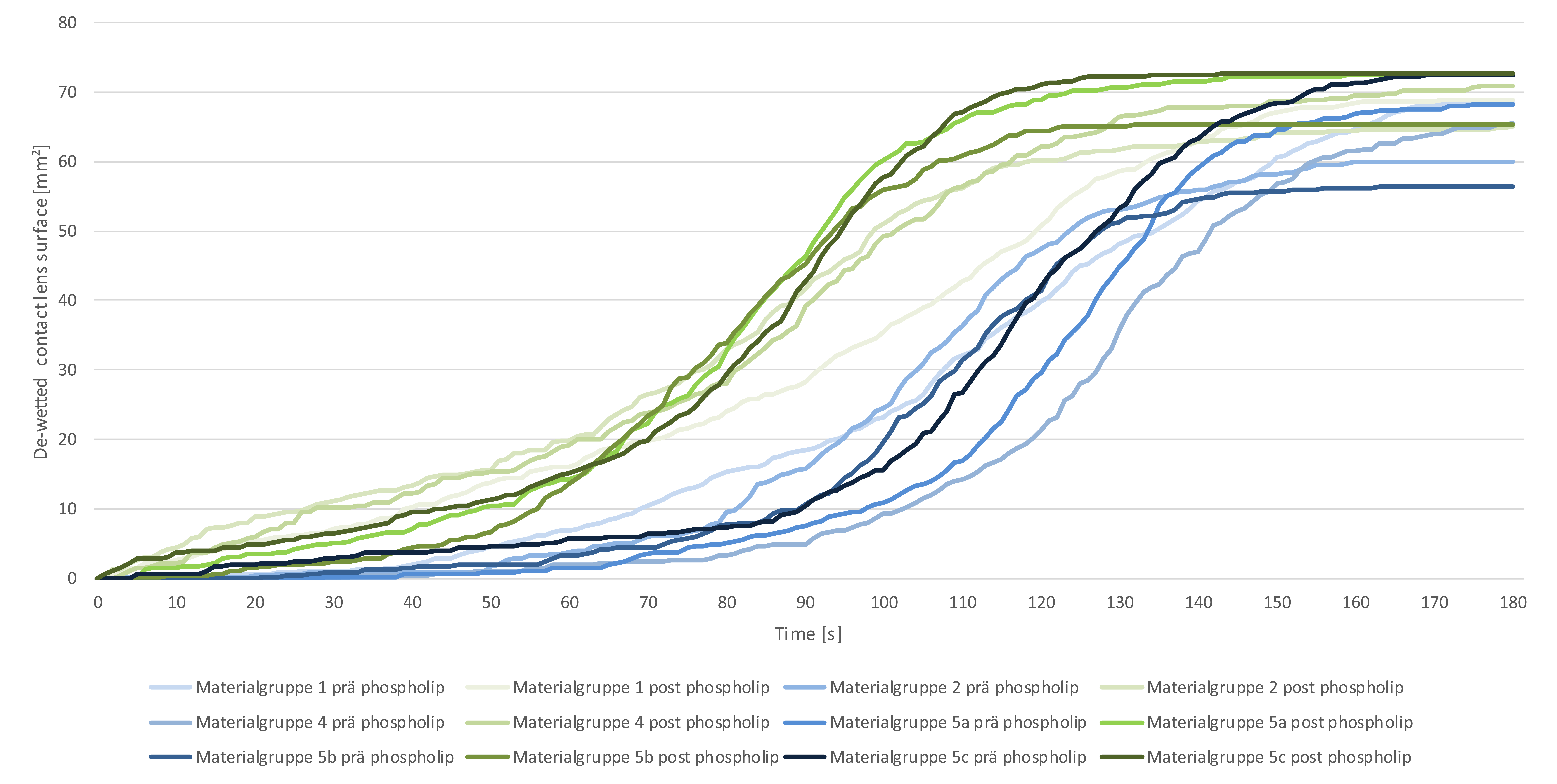
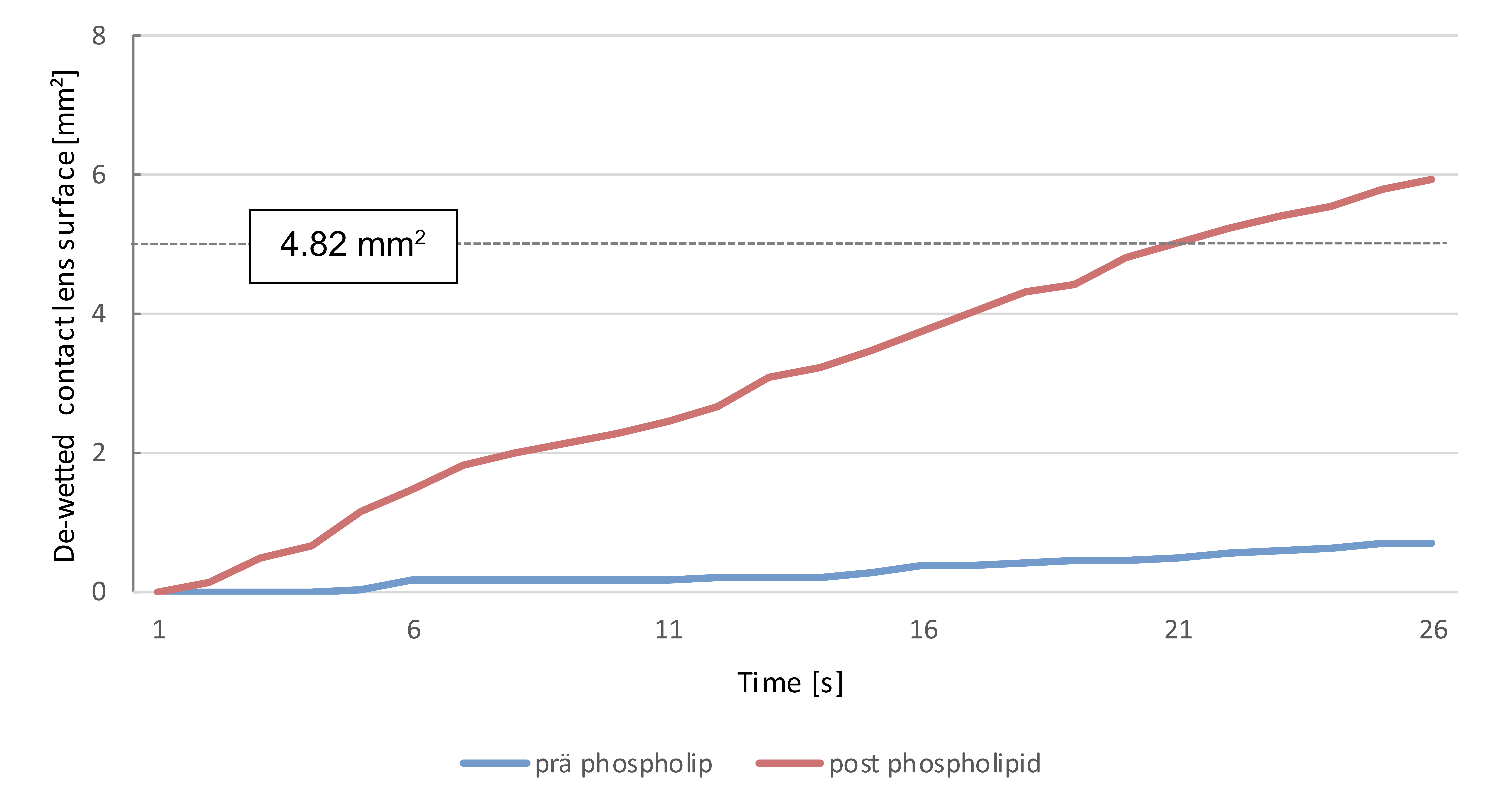
Discussion
The measurement results show that the drying rate of a thin moisture film depends on liquids previously used for storing the CL. This has also been observed in other in vitro studies.33,31De-wetting is influenced by several factors, including evaporation, surface structure, temperature, humidity and gravity. Three primary factors, i.e. the adhesive forces, the cohesive forces and the surface tension, have a decisive influence on the de-wetting process. These decrease with increasing temperature and are higher with larger molecular surfaces.39 In this study, all measurements were carried out at a room temperature of 21°C and 45% humidity; conditions also reported in comparable studies.32 Since the thickness of the layer and the environmental conditions differ with regard to an in vivo situation, the break-up times and the subsequent de-wetting phenomena cannot be compared one-to-one with the clinical practice.31 It was possible to show that the constituents of the phospholipid-containing test solution interacted during the storage period as a result of the movement of these constituents over the lens surface. This can be seen from the fact that, despite renewed equilibration in saline solution, a significantly faster de-wetting of the CL was measured after being stored in the phospholipid-containing solution. This was the case with all contact lens materials examined and is reflected by the primary target variable. The AUC value increased by an average of 53% in the group of hydrogel materials and by an average of 54% in the group of silicone hydrogel materials. Although experience has shown that silicon hydrogel materials are more lipophilic,24 it was in particular the hydrogel materials, the ones that exhibited accelerated de-wetting after being placed in the phospholipid-containing solution, since they reached the secondary target variable earlier. On average, the time required to achieve 4.82 mm2 of de-wetted surface was 68 s in the case of the hydrogel materials and 69 s in the case of the silicon hydrogel materials, which, after being kept in the phospholipid-containing solution, was shortened to 15 s and 31 s respectively. This corresponds to a 78 % acceleration in de-wetting in the case of the hydrogel lenses and 55 % in the case of the silicon hydrogel lenses. It can be assumed that further constituents of the phospholipid-containing solution accumulate on the surface. Since no subsequent investigations have been carried out in this respect, this, however, cannot be proven.
Retrospective studies and clinical publications show that the composition of the phospholipid-containing solution used is complex and that the product is well accepted by users.40,41 The effect of ectoin on the natural lipid layer and in combination with artificial lipids shows that it has a promising potential as a treatment for dry eyes.42,43 In particular, it improves the fluidity of the lipid layer, which supports the superficial linking of the lipids.43 Whether this can be advantageous for symptomatic contact lens wearers remains the subject of current clinical studies. First indications however imply certain benefits20. These clinical studies are important because experimental laboratory investigations cannot accurately reflect the clinical situation. Factors such as friction during blinking, the change between de-wetting and re-wetting of the contact lens surface or the eye temperature can only be imitated to a limited extent in the laboratory and can lead to different results in reality. Since the phospholipid-containing eye drops dilute in the lacrimal fluid after application, the amount of phospholipid which interacts directly with the contact lens surface is likely to be significantly lower in an in vivo situation. Changes in the contact lens surface relevant to visual acuity occur only after more than 10 s after the blinking.44-47 It can thus be assumed that the use of phospholipid-containing eye drops does not lead to a decrease in visual acuity level by one log level within this time period.
Conclusions
It can be demonstrated that phospholipids lead to faster de-wetting when interacting with a contact lens material for a prolonged period of time. As the de-wetting area of 4.82 mm2 is reached by contact lens wearers after a typical interblink period during visual tasks requiring a certain level of attention, it is unlikely to expect a drop of the visual acuity by one log level.
Conflict of interest
The authors declare that there is no conflict of interests regarding the methods and devices mentioned in the article.