Intraocular lenses for patients with age-related macular degeneration
Purpose. Age-related macular degeneration refers to a retinal disease that induces the loss of central vision and is the most common cause of blindness in people over 65 years of age in the western world. Today’s technology offers various intraocular lenses, which are used to support conventional low vision aids such as collimating and non-collimating magnifiers.
Material and Methods. Within the scope of this thesis, three different intraocular lens systems are presented, which are based on the principle of beam deflection and/or magnification.
Results. Following a patent and literature search, the systems were simulated using ray trace software in order to reconstruct their functional principle and compare it with each other.
Conclusion. With the help of a theoretical model, different fictional retinal defects were simulated and the efficiency of each lens system was evaluated according to their respective properties.
Introduction
Age-related macular degeneration (AMD) is an eye disease that leads to a continuous and often unnoticed loss of vision.
As the disease progresses, the individual‘s threshold of perception is exceeded at some point, so that affected people consciously perceive the visual impairment. In some cases, not only the disease stage changes, but also the form of AMD and the creeping process of vision loss changes into a rapid deterioration in vision.1 The disease affects central retinal region (the macula) or more precisely the point of sharpest vision (the fovea centralis).
AMD causes stadium-dependent, polyvalent or less pronounced central field of view (scotomas), which results in blurred and distorted perception of fixed objects or their total unrecognizability.2
Untreated, the disease leads to complete blindness in some cases.
The progress of AMD can be slowed down and ideally even stopped with the help of different therapy approaches, but it is currently not possible to restore vision that has already been lost.
For this reason, AMD patients are permanently dependent on special visual aids in order to be able to perform activities at different viewing distances with reduced restrictions. Enlarging visual aids in the form of magnifying glasses (collimating or non-collimating) or screen readers, as well as specially manufactured intra ocular lenses (IOL) are used to support therapy. The primary objective of the research is the further development of medical treatment approaches and supplementary visual aids, especially in the field of intra ocular lenses, in order to give AMD patients a certain amount of independence in everyday life.
In this publication, the focus is on raytracing simulations of existing IOL concepts for patients with AMD. The simulated systems are reduced to the essential aspects in order to provide an assessment of the retinal images obtained. For this purpose, the positions and various sizes of optotypes are used and set in relation to fictitious scotomas.
Materials and Methods
hree different IOL systems for patients with AMD were transferred into a model for raytracing simulations using a commercially available software (OpticStudio, Zemax LLC, USA). An eye model according to Liou & Brennan 3 provided by Zemax was used for the simulations of the systems presented below. It is described as a so-called „finite eye model“ and has three aspherical surfaces and a gradient index lens. The total optical power is approximately +61 D with an axial length of 24 mm. It is an anatomically, optically and biometrically realistic eye model that offers the possibility of calculating optical transfer functions and predicting the performance of the eye. The Liou & Brennan model serves as the basic system, which was modified for the simulations of the IOL systems. Since the following simulations refer to pseudophakic eyes, the Liou & Brennan lens data were replaced by corresponding IOL data.
In addition to the Liou & Brennan eye model, other biometric realistic eye models exist, such as the Navarro eye model or the Arizona eye model.4 As the focus of the work is on the comparison of the different intraocular lens systems, these were not additionally implemented.
All simulations were carried out for a monochromatic wavelength of 550 nm in the sequential mode of the raytracing software, which is designed for the simulation of monofocal lens systems.
The Scharioth macular lens (SML)
Since the end of 2014, an add-on IOL for the treatment of advanced dry AMD has been available on the market, which is primarily designed to enhance visual acuity in the near field. The Scharioth Macula Lens (short: SML, 1stQ GmbH, Germany) is a foldable hydrophilic acrylic lens (refractive index 1.461), which was developed for implantation into the sulcus. The bifocal IOL has a total diameter of 13 mm and has four flexible haptics. The optically effective area of the concave-convex lens measures 6 mm in diameter and consists of a central, 1.5 mm large addition part of +10 D and an optically neutral edge area. The one-piece add-on IOL is designed for a monocular implantation for pseudophakic eyes and is implanted into the better seeing eye. According to the manufacturer, the peripheral visual range, due to the optically neutral edge area, remains unimpaired for distance and a standard visual distance in the near range (approx. 40 cm) and binocular vision. Only at a reading distance of approx. 15 cm foreseen for the SML, one makes use of the fact that binocular vision is restricted and the image information of the other eye is faded out by the brain, whereby double images are avoided. Due to the high addition of the central area, the SML acts like a magnifying glass and produces an approx. 2.0x magnification of viewed objects in the near distance. A central scotoma is thereby artificially reduced in size by magnifying a fixed object.5,6,7,8
The Fresnel lens
In 2014, an article on a pilot study was published in the Journal of Cataract and Refractive Surgery in which the results of an implanted defocusing capsular bag IOL were presented.9 It is a stable PMMA-IOL (refractive index 1.49) which has a prismatic Fresnel structure on the rear surface of the lens, whereby incident light is focused on parafoveal retinal areas. With the help of this intra ocular lens, which was designed for the correction of distant vision aphakic eyes and has an optical power of + 20 D, the shift of the image to a peripheral retinal region not covered by a of a centrally located scotoma is possible. The back-surface prism structure produces an image deflection of about 6°, whereby retinal decentration of 1.8 mm is realized on the retina. This information referred to in the article refers to an ocular axis length of about 23.1 mm and a monocularly implantation into the better
seeing eye.
The iolAMD lens-system
The iolAMD (London Eye Hospital Pharma, UK), consists of two hydrophobic acrylic lenses and is based on the mode of action of a Galileo telescope.10,11,12 The two-lens system is designed for implantation in aphakic eyes, whereby the biconvex lens is inserted into the sulcus and the biconcave lens into the capsule bag. The optical power of the sulcus lens is about +63 D with an optical diameter of 5 mm and a total diameter of 12 mm. The sulculs lens has hyperbolic front- and back-surfaces and is decentered vertically with respect to the central optical axis of the whole eye by 0.85 mm. Due to additional curvatures of the haptics, the sulcus lens is shifted in direction of the iris. The second IOL is located at a distance of 2 mm from the sulcus lens, with an optical power of about −49 D and total diameter of 11 mm, the optic diameter is 4 mm. The haptic design of this lens is symmetrical and has an angulation of about 15°, which shifts the IOL backwards to ensure the distance between the two lenses. Both lenses have a refractive index of 1.525 and in combination produce an overall optical power of approximately +18.5 D. The lens system deflects incident light by about 3° (= 0,87 mm) from the center of the foveola and simultaneously enlarges the retinal image by about 1.2 times.
Results
The SML
Within the simulation of the SML, the provided eye model based on Liou & Brennan 3 was modified by a hydrophilic reference IOL and an aspheric add-on lens. According to the literature, the sulcus lens consists of hydrophilic acrylate and has an optical power of +10 D, at a diameter of 1.5 mm.13 Since the rest of lens area is optically neutral according to literature, the simulation of the edge area was omitted, so that the total diameter of the add-on lens is also 1.5 mm. The side view of the lens system shows that all simulated rays outside the sulcus lens diameter, in sequential mode, do not contribute to image formation. By hinting at the extended front surface radius of the add-on IOL (dotted line) it is to be seen that the beam representation ends along this imaginary line (figure 1b).
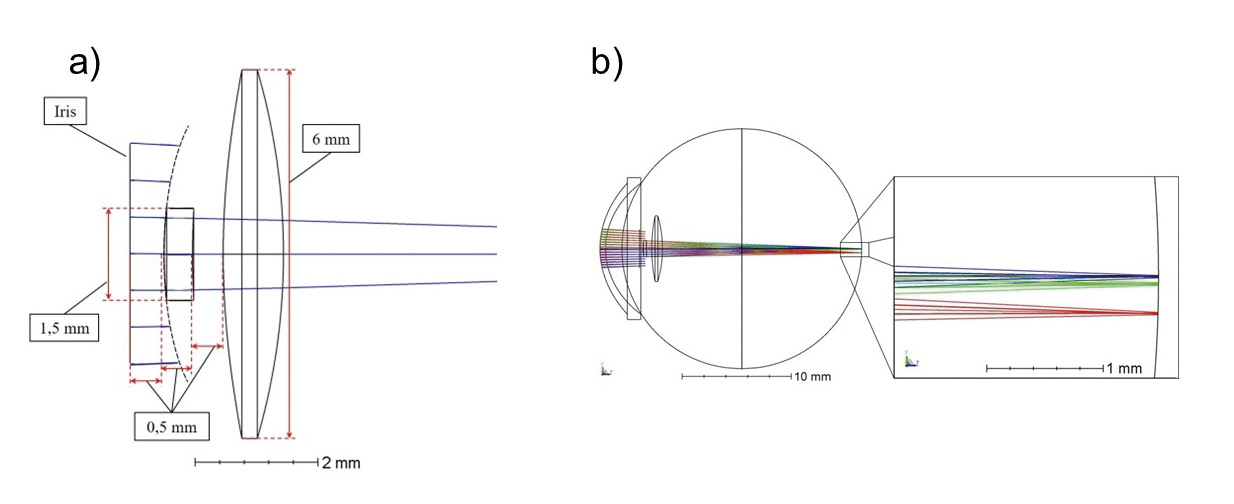
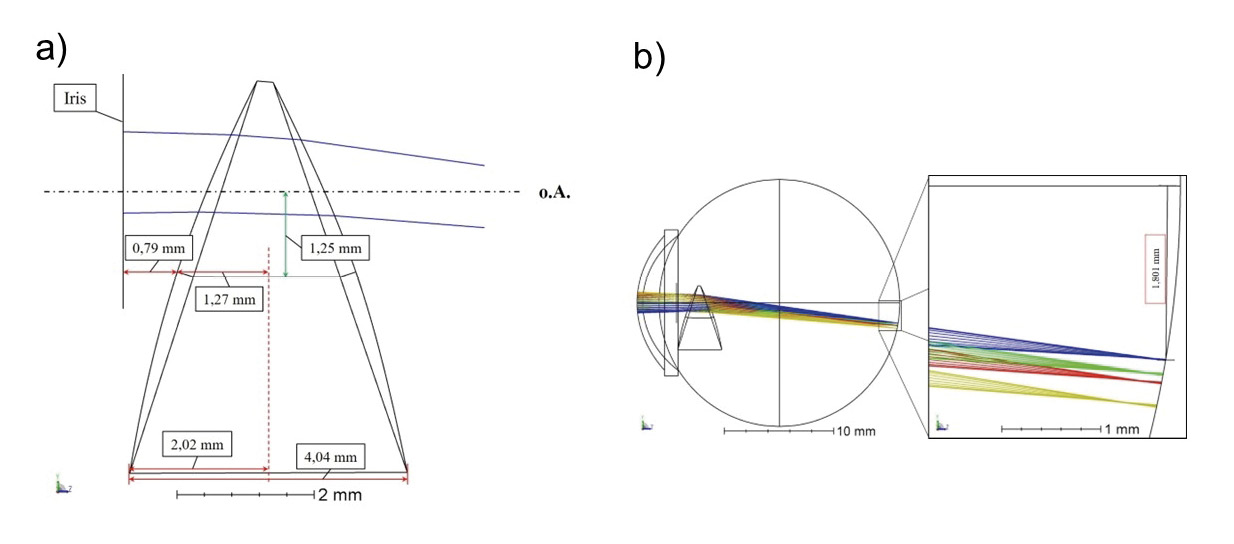
The add-on lens is located at a distance of 0.5 mm from the front surface of the iris and lens and features an aspherical front surface to improve image quality. The lens system produces a magnification of approx. 2.1 times. As the lens is intended as low vision aid for near range, an object distance of 15 cm was selected for the simulations.
The Fresnel-Lens (Prism-System)
In order to reproduce and simulate the mode of action of the intraocular lens, the Liou & Brennan eye model 3 was simplified by omitting the complete Fresnel structure and creating only a wedge prism with two radius surfaces that achieves the desired deflection angle. The main focus was the reproducibility of literature results,6 wherefore a possible implantability of the IOL was not relevant and was not taken into account in the shaping process. The angulation of the anterior and posterior surfaces of the lens was accordingly selected in such a way that the decentering of the retinal image of 1.8 mm is achieved. Consequently, the first refractive surface was tilted by +18° and the second refractive surface was tilted by −19°, each around the x-axis. This results in a prism base length of 4.04 mm with a lens diameter of 6 mm (figure 2a).
In addition, both sides of the PMMA lens were provided with conical radius surfaces to increase the convergence of incident ray bundles and to produce a sharp image on the retina. The optical power of the IOL is therefore +20 D. In order to produce a total shift of the retinal image of 6.2° (= 1.8 mm), the prism wedge for the simulation was additionally decentered by 1.25 mm perpendicular to the optical axis so that incident light propagates through the upper prism part (figure 2b).
As the deflection angle increases with increasing distance to the base, the required image deflection could be realized by means of wedge centering without changing the refractive index or the optical effect of the IOL. A shift of the prismatic Fresnel lens normally is not planned.
The deflection angle of the simulated prismatic system amounts to 5.9° for an on-axis objective point in distance of 6 m.
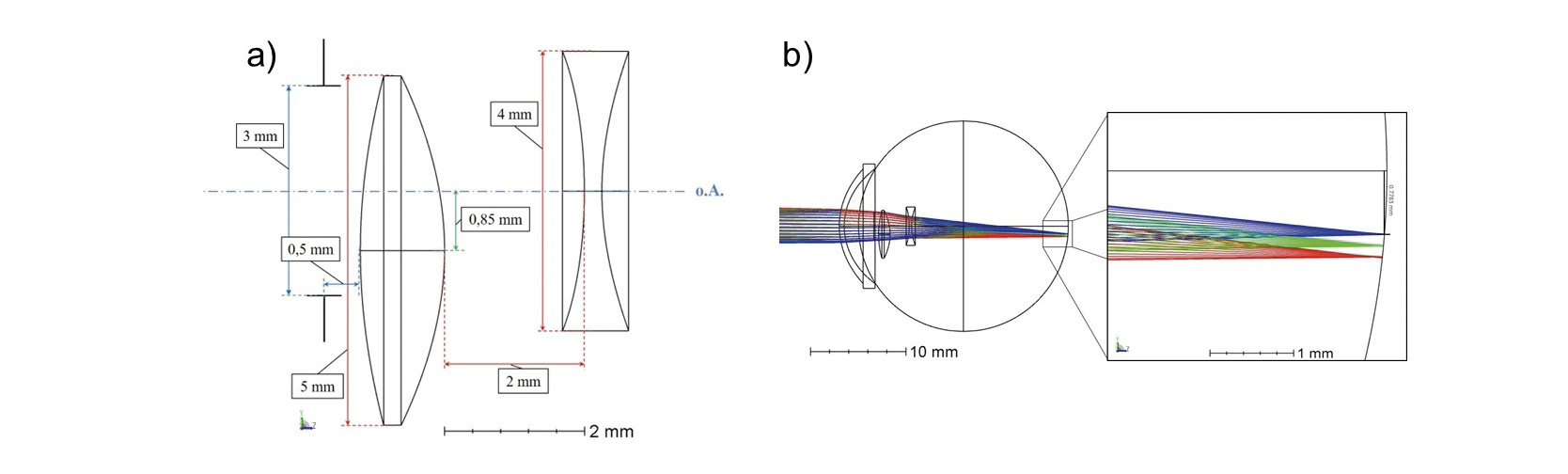
The iolAMD-System
In the simulation of the two-lens system, the Liou & Brennan eye model 3 was modified by a biconcave capsular bag IOL, a biconvex sulcus lens and a spectacle lens correction. Both IOLs are made of hydrophobic acrylate with a refractive index of 1.525 and have conical front and rear surfaces (figure 3a).
By adding a spectacle lens, which has an optical power of +3 D and a refractive index of 1.517, the emmetropic eye model was modified into an hyperopic eye model. This allowed more degrees of freedom in the simulation for optimization of the objective IOL (sulcus lens) and ocular IOL (capsular bag lens) radii for an object distance of 6 m, as the conic parameter are unknown. Furthermore the dioptric power of the simulated lens system is in the range of the commercially available power. The plano-convex spectacle lenses each have a distance of 12.5 mm to the corneal vertex.
The biconvex lens has a 5 mm diameter and is located 4.72 mm behind the anterior surface of the cornea. The objective IOL was decentered by 0.85 mm perpendicular to the optical axis inferiorly, whereby a retinal image shift of 2.7° (= 0.78 mm) was achieved. The capsular bag IOL, whose diameter is 4 mm, is located on the optical axis at a distance of 2 mm from the sulcus lens. To avoid scattering effects at the upper edge of the biconvex lens, the pupil diameter has been reduced to 3 mm. The lens system provides an optical power of about +25 D and a magnification of 1.297.
Two decimal visual values were simulated for the far distance and near distance. Both systems included the above mentioned spectacle correction of +3 D for a 6 m distant object and +5.5 D for a 40 cm distant object.
Comparison of all systems
In the following, fictitious retinal defects are illustrated in combination with images of the tumbling E of the individual lens systems.
For this purpose, some of the already known retinal images of corresponding visual acuity 14 values are used and shown to scale in a schematic retina model, in order to illustrate the mode of action of the systems and to get an idea of the dimensions of image and retina measurements, as well as the scotomas. For the foveola a diameter of 350 µm is assumed, for the foveal avascular zone (FAZ) 600 µm and for the fovea 1.5 mm.
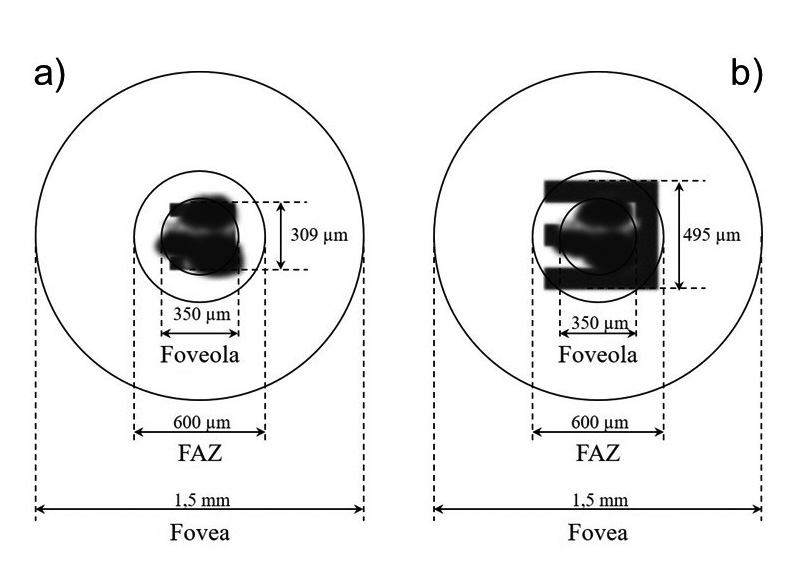
The sketches in figure 4 show dimensions of the retinal areas in simplified, circular geometry. Both models show the geometric retinal images generated by the SML-System and correspond to the image sizes of the visual values 0.125 (figure 4b) and 0.2 (figure 4a). Both images show a retinal defect of the same size which extends over the diameter of foveola.
The selection of this images is conditioned by the choice of fictitious retinal defect. This visual acuity is mentioned in various publications as the lowest postoperative refractive result and corresponds to an image size of 309 µm on the retina. An image of this size is not visible in case of a defect affecting the whole foveola.
Since the highest postoperative refractive result after implantation of the SML was 1.0 and this value corresponds to a retinal image size of less than 70 µm, this result was not shown. Instead, in figure 4b a lower visual level and therefore a larger retinal image has been selected for clarifying the dimensions. Illustration shows that the tumbling E has a size of about 500 µm and exceeds the extent of the retinal defect, allowing to identify the openings of the tumbling E.
The depicted retinal defect is such extensive in the model, that the visual acuity values known from the literature and the associated image sizes are not recognizable.
By clarifying the mode of action of the prismatic system from figure 5 the same retinal dimensions were represented in a simplified way and extended by the range of parafovea.
The system generates a beam deflection of approximately 6° from the center of the foveola, which corresponds to an image shift of 1.74 mm on the retina and thus exceeds the radius of the parafoveal region. The retinal images shown correspond to the visual levels and have been adapted to the scale of retinal dimensions for the model shown here. Based on the listed image sizes, it is evident that the images of the visual values 0.1 (245 µm) and 0.16 (154 µm) cannot be reached without the distraction generated, even for a 350 µm retinal defect in the center of foveola (figure 5a). With a scotoma of size 1.5 mm, which corresponds to the dimensions of the fovea, it can be seen that none of the geometric image simulations shown could be resolved in the center of the retina (figure 5b).
The sketch thus illustrates the advantage of the prismatic system by deflecting the ray beam, which shifts the retinal defect away from the visual center to the edge of the visual field. At the same time, the image shift to perifoveal retinal areas means a reduction of the uptake/resolution capacity, as the receptor density outside of the fovea decreases sharply. For a beam deflection of 6°, the retinal output decreases to a decimal visual value of about 0.4 compared to the center of the foveola, where the visual acuity of 1.0 is attainable with a healthy eye.15 Local visual performance causes an additional reduction in the image quality of produced retinal images, which is expressed in the form of low-contrast images. This effect was not shown in the sketches due to the lack of visual data on this contrast reduction.
The models in figure 6 show the dimension of geometric images and retinal areas from foveola to parafovea created by the iolAMD -System. The resulting image sizes correspond to the 0.1 and 0.2 visual acuity values known from the literature, which are indicated as the lowest and highest postoperative refraction result. The two-lens system generates a beam deflection of about 3° by decentering the first lens, which corresponds to an image shift of 0.87 mm on the retina.
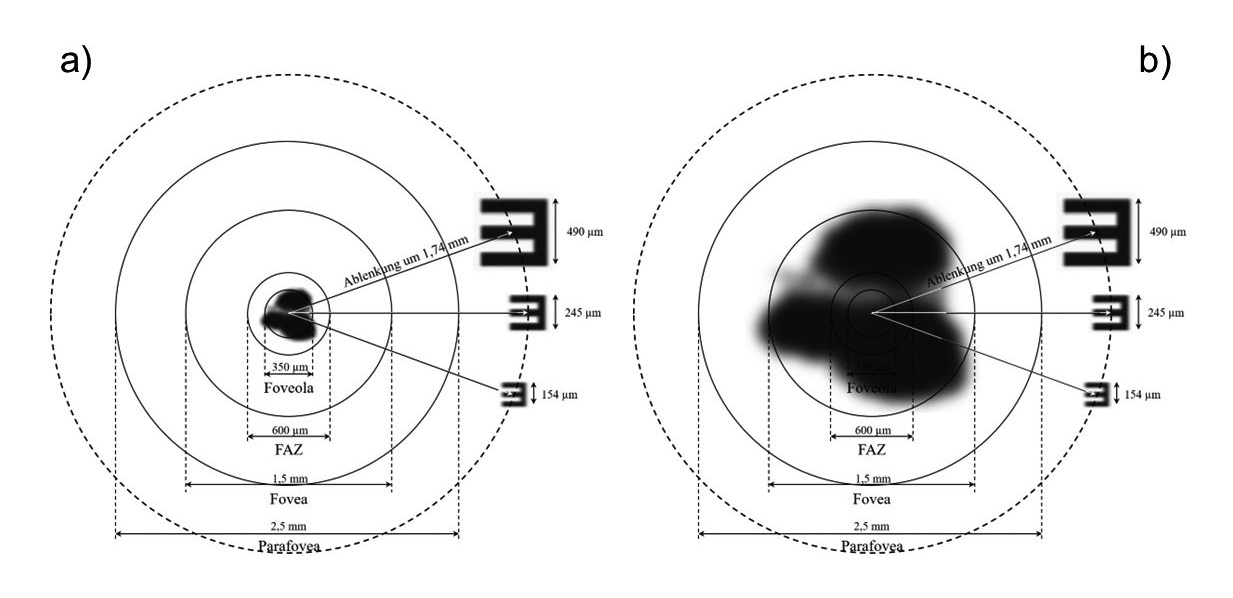
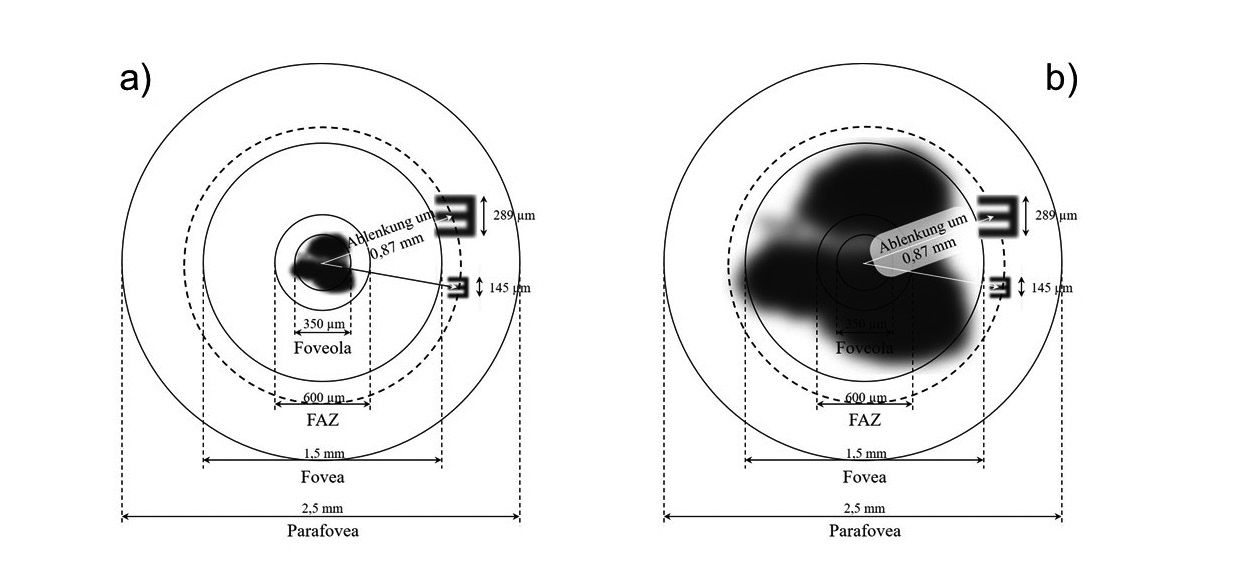
As a result, the retinal images are located in an area outside of the fovea, making the tumbling E visible even when the scotoma is 1.5 mm in diameter (figure 6b). For a non-shifted two-lens system, where the retinal images are located in the center of the foveola, it can be seen that the image sizes shown here are not recognizable, since an image of the visual acuity 0.1 is smaller than the retinal defects shown.
The advantage of decentering the image is therefore the decimal visual acuity that can be achieved, which is helpful in relation to the reduction in visual acuity due to scotoma expansion. As a disadvantage, the reduced maximum retinal resolution within the parafoveal region, which, due to a reduced receptor density, lies at a decimal visual value of about 0.55, is to be listed.13 As with the Fresnel-lens (Prism-System), this implies a reduction of contrast vision, which is less pronounced here due to the lower image shift. This effect is not shown in the sketches shown here, as there is no visual data on this contrast reduction.
Discussion
The schematic representations of all geometric images for different retinal defects serve to visualize the image formation on the retina by the different lens concepts and are subject to the data sets obtained from the simulation. A limitation of these simulations is, that a realistic representation of the geometric images was not possible because the simulation did not influence the contrast reduction due to the beam deflection of the prismatic and two-lens system. However, the respective dimensions with regard to image size and retinal areas were feasible, which also revealed the effects of differently pronounced retinal defects.
The SML-system has good imaging properties, but the postoperative visual acuity levels have been achieved in patients with a lower retinal defect than the fictitious one shown here.7,8 If a scotoma of the size of the foveal center were present, these high visual acuity values would not be attainable. The advantage of the system compared to the other two IOLs, however, is that the generated image is mapped to the central region with the highest retinal performance, whereby the area with the comparatively highest receptor density is used in the case of minor retinal defects.
The situation is different with the Fresnel-lens (Prism-System), which generates such a large beam deflection that even large central retinal defects, from the patient‘s point of view, are pushed to the edge of visual field. Disadvantages of the system are, however, above all the reduced retinal performance, which is equivalent to a visual acuity of 0.4 due to the comparatively low receptor density and the main presence of rods in perifoveal areas of the retina.
The clear advantage of this system is that even patients with a large retinal defect are able to see at a distance and close range. For those affected, this means a significant improvement in their quality of life for the new learning of vision, which is inevitably associated with this, due to the alternative visual range.
A good compromise system is the iolAMD lens, which combines the optical advantages of both previous systems by combining magnification and beam deflection. Although there are fewer receptors in the parafoveal areas of the retina and proportion of cones is clearly reduced in comparison to the visual center, the retinal performance, however, is 3° away from the foveal center, equivalent to about 55 %. By shifting the two-lens‘ main plane position and using the +3 D spectacle correction in the simulation process for adjustments, the resulting retinal image is already magnified at a distance, compared to a monofocal IOL.
With appropriate add power, this effect can also be transferred to the near distance. In view of the achieved postoperative visual acuity values, patients achieved on average less success with this lens system than with the SML, but this can also lead to the shift of pronounced retinal defects to the edge of the visual field. The adjustment and new learning of vision with the iolAMD lens is subject to similar requirements as with the Fresnel-lens (Prism-System).
A strict observance of the distance between the two lenses is probably one of the system‘s weaknesses, since any deviation from the specified lens distance has a detrimental effect on the image quality in the raytracing process and this distance might be subject to a certain tolerance during the implantation of both lenses.
Conclusion
The aim of this work was to compare image simulations of different intraocular lens systems, which are designed for patients with age-related macular degeneration, in a schematic eye model.
Three systems were selected, which were simulated by means of raytracing software and their mode of operation was simulated by means of different evaluation diagrams.
The simulated lenses are a centrally enlarging system based on the SML, a deflecting system based on a prismatic Fresnel-lens and a decentered lens system based on the principle of iolAMD lens.
The main requirement of this work was the literature-based, best-possible design of the systems in order to clarify the functionality and determine the image quality of each system.
It was possible to create several geometric images for each lens system, which were used for the comparison of all systems on the basis of the Liou & Brennan schematic eye and a simplified schematic retinal model for scotoma.
With the help of the created model, it was shown how the beam shaping and guidance of each system, in combination with fictitious retinal defects of different sizes in the central retina area, has an effect.
In consideration of various factors, a final evaluation was possible in comparison of all systems. The result is that the choice of IOL depends mainly on the stage of the disease.
For small central scotomas, the SML is well suited in the simulations to achieve visual acuity values suitable for everyday use at a distance of 15 cm. For larger scotomas that exceed the fovea, however, its use appears less appropriate. Here, the deflecting systems (Fresnel-lens and iolAMD) seem to offer better options, even if the resolution of the retinal cells is reduced peripherally.
Conflict of Interests
The authors declare that there is no conflict of interests regarding the methods and devices mentioned in the article.
Degeneration. Med. Clin. North Am., 105, 373-491.